Saliva is far more than an occasional nuisance ‒ the embarrassing, watery drool that drips off your lower lip when you least expect it. Saliva is a complex, multi-purpose secretion that originates within salivary glands located in the oral cavity, and the composition consists of 99% water. The remaining 1% is composed of many organic and inorganic molecules. A few examples of the 1% portion include calcium, phosphorus, amino acids, mucoids, salivary enzymes, hormones, and proteins.1
Three major paired salivary glands secrete about 90% of saliva. The parotid gland contributes about 20%, with most of it being serous, watery, and thin secretions of high inorganic content (calcium, bicarbonates). The submandibular gland contributes around 65%, consisting of a mixture of serous and mucous secretions. The sublingual gland contributes about 5 to 7% of mostly mucous, more viscous secretions. The remaining 10% comes from hundreds of minor salivary glands (labial, buccal, and palatine, along with anterior and posterior glands) distributed throughout the oral cavity.2
This article gives us a brief overview of the anatomy of the salivary glands, then a review of some of the important functions of saliva related to digestion and speech. The article also details some of the saliva’s many protective properties within the oral cavity.
The Major Salivary Glands
The largest of the three pairs of major salivary glands are the parotid glands. They’re located on the sides of the face just below and in front of the external ears. Each of them is about the size of a small-to-medium size pepperoncini pepper and weighs just over 14 g. They produce about 10% of the mouth’s saliva when not eating, but that number increases to about 25% when stimulated by eating.3
The serous saliva produced contains the enzymatic protein amylase that begins the process of digestion in the mouth. Amylase converts starch and glycogen into simple sugars. Each gland empties saliva into the mouth through a duct known as the Stensen duct. It is about 5 cm long and exits from the anterior border of the parotid gland (much like the stem exiting pepperoncini) and opens into the mouth through the buccal mucosa opposite the 2nd maxillary molar.4,5
The submandibular glands are located alongside and just below the mandible. They are the second largest of the three major salivary glands. Each is about the size of a walnut and weighs about 8 to 10 g. They contribute the majority of the unstimulated salivary flow to the mouth. Each secretes into a duct about 5 cm long, known as Wharton’s duct. Wharton’s duct traverses forward and empties into the floor of the mouth on either side of the lingual frenulum, adjacent to the sublingual caruncle.6
The sublingual glands are the smallest of the major salivary glands. About the size of an almond, each weighs about 4 to 5 g. They are located under the floor of the mouth and below either side of the tongue.7 The sublingual gland itself is composed of a major sublingual gland and eight to 30 small, minor sublingual glands. The sublingual duct, known as Bartholin’s duct, drains the major sublingual gland into Wharton’s duct, while multiple tiny ducts called the Ducts of Rivinus drain the minor sublingual glands into the floor of the mouth.6
The Minor Salivary Glands
Hundreds of minor salivary glands exist throughout the aerodigestive tract (the combined organs and tissues of the respiratory tract and the upper part of the digestive tract).7 Most of them are too small to be seen without a microscope. They are dispersed throughout the submucosa of the sinonasal cavity, oral cavity, pharynx, larynx, trachea, lungs, and middle ear cavity. Most are concentrated along the buccal mucosa, labial mucosa, lingual mucosa, soft/hard palate, and floor of the mouth.6 The gingiva and the hard anterior palate don’t contain any minor salivary glands. Each gland is small, typically 1 to 2 mm in size.
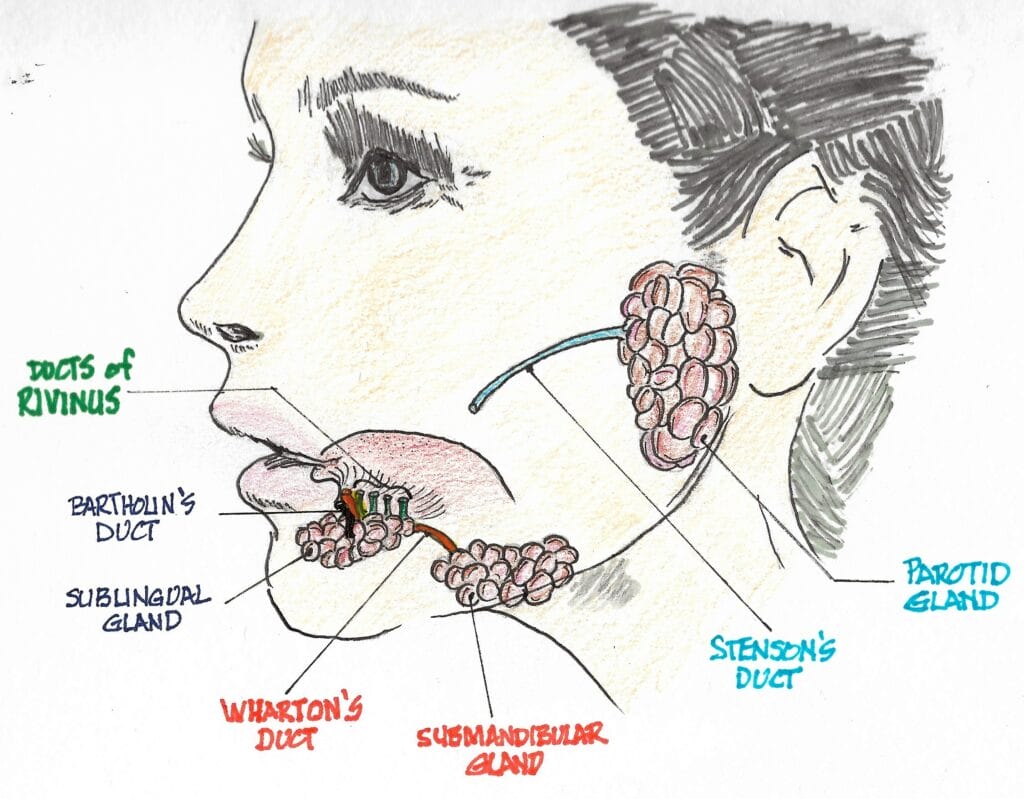
Newly Discovered Salivary Glands?
In early 2020, researchers in the Netherlands were testing prostate and urethral gland cancer patients using prostate-specific membrane antigen – positron emission tomography/computed tomography (PSMA PET-CT) scans.8,9 These scans look for areas where the PSMA protein is found, such as the surface of prostate cancer cells, but also in healthy salivary glands, which also have high PSMA-ligand uptake. These areas will overexpress the area and show up as a glowing image on the test screen.
They found that all their patients tested positive for the PSMA protein in a 4 cm long area of the nasopharynx known as the torus tubarius (see Figures 2 and 3). The researchers labeled these glands atop the torus tubarius as the tubarial salivary glands.
The torus tubarius is the cushion of the auditory canal. It is a mucosal elevation of the nasopharynx (the uppermost part of the pharynx, located behind the nose) with the eustachian tube opening immediately anterior to it. This bilateral area of predominantly mucous glands with multiple drainage ducts appears to indicate characteristics of salivary gland structure. Ongoing research will determine whether these are indeed salivary glands and, if so, whether they are major or minor salivary glands. The research will also show details as to their exact functions.
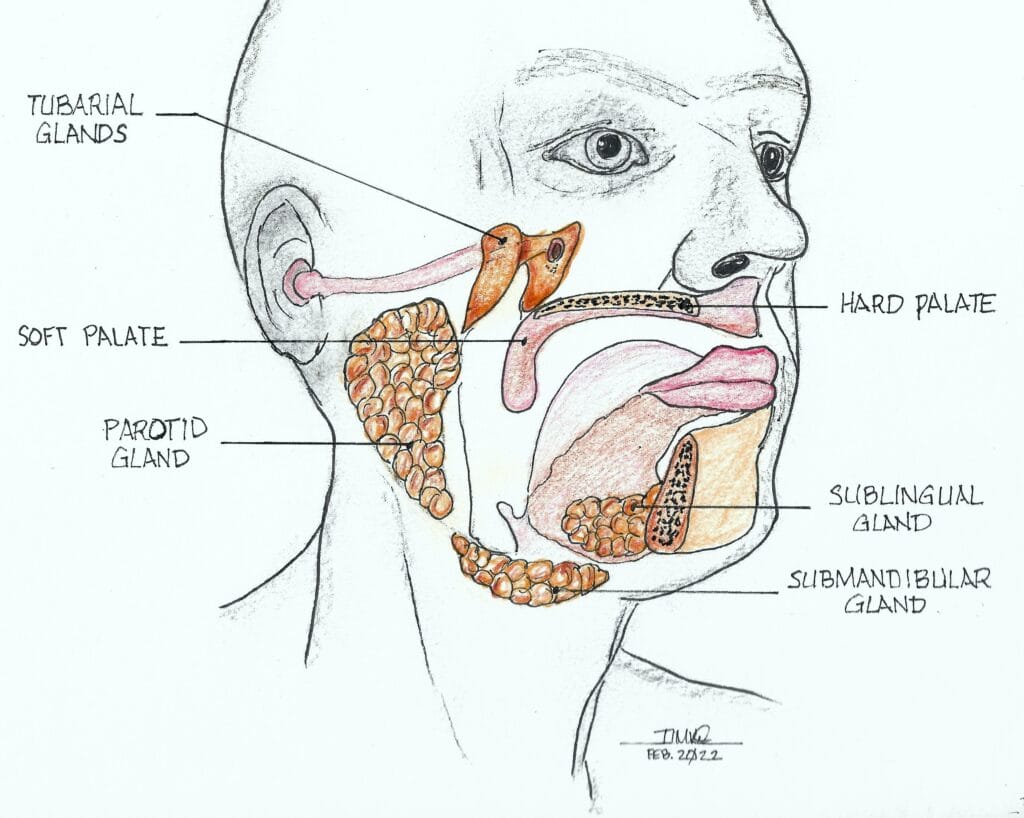
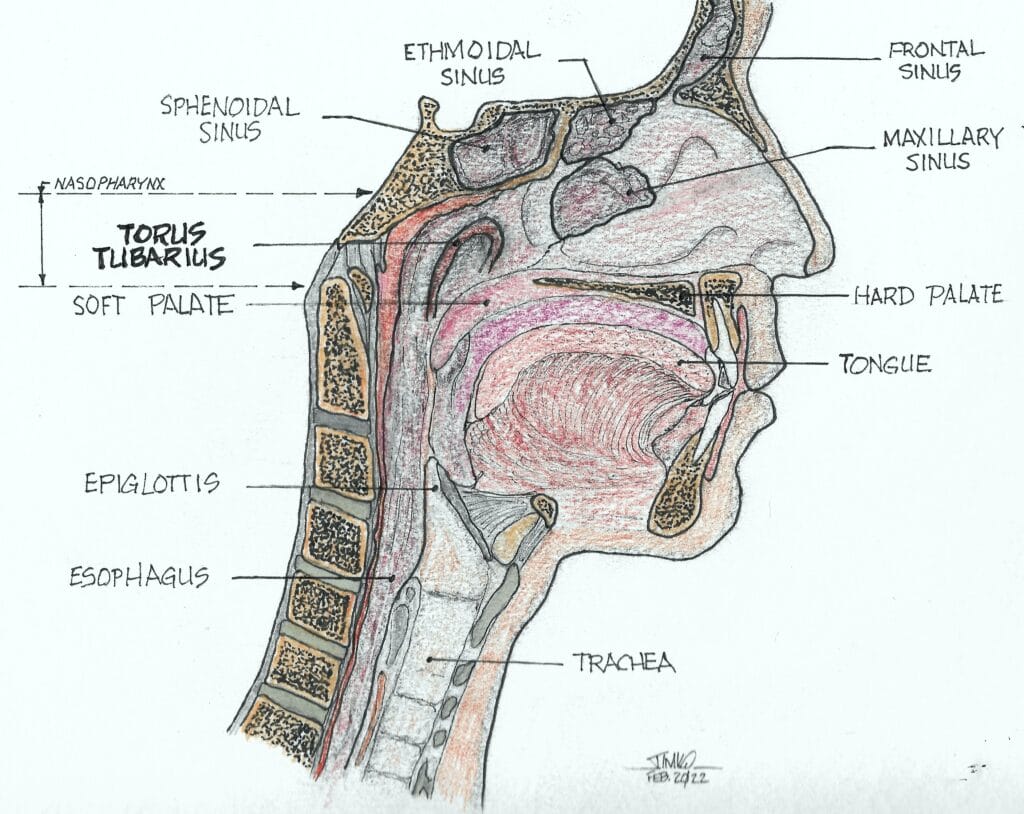
Salivary Flow Rate
The normal daily production of saliva for an adult varies between 0.5 and 1.5 liters.10 If we use a median value of one liter per day of saliva being made, that means your salivary output will fill a four-person 300-gallon hot tub with spit every 3.1 years! That’s a lot of salivary output for three small major and many hundreds of minor salivary glands.
The unstimulated saliva flow rate (no exogenous or pharmacological stimulation) is approximately 0.3 to 0.4 ml/minute. This rate decreases to 0.1 ml per minute during sleep and increases to about 4 to 5 ml per minute during eating, chewing, and other saliva stimulating activities.10 Salivary gland secretion is controlled by the autonomic nervous system.
Parasympathetic stimulation produces abundant quantities of watery saliva, whereas sympathetic stimulation produces more viscous saliva. Sympathetic stimulation also dominates during times of stress, resulting in a dry mouth. During dehydration episodes, the salivary glands stop secreting to conserve water.
Xerostomia (dry mouth) is a common oral problem associated with some 400 to 500 medications. Polypharmacy is the most common cause of xerostomia. The most common types of medication with xerogenic potential are those with anticholinergic and sympathomimetic actions. Anticholinergic agents block the action of acetylcholine, a neurotransmitter. Antisympathomimetic agents inhibit the action of epinephrine and norepinephrine.
Salivary gland hypofunction is a condition often overlooked. Many patients who take xerogenic medications do not know that they’re at risk of oral complications such as dental caries, fungal infections, and difficulty in wearing dentures.1,10 Other causes of xerostomia include autoimmune diseases such as Sjögren’s syndrome, head and neck radiation therapy, diabetes, or an oral yeast infection.1 Typically, if the flow rate falls to less than half the normal flow rate, it is considered xerostomia. The salivary flow rates for xerostomic patients typically drop to somewhere between 0.1 to 0.2 ml per minute.
Digestion Begins in the Mouth
Saliva is an essential component of the digestive process. Since saliva is 99% water, it moistens the mouth and helps convert whole foods into softened particles for easier swallowing. Our teeth and tongue work much like a food processor, using saliva as the liquid necessary to create a mixture of food (bolus) ready for the stomach to continue the digestive process.
Saliva contains the enzyme amylase, which breaks down starches (complex carbohydrates) into sugars within seconds of digestion, which your body can absorb more easily.10 The majority of amylase is made in the pancreas. It also contains an enzyme called lingual lipase, which breaks down fats. Saliva helps both of these digestive processes to begin in the mouth.
Some of my most effective educational discussions with my patients regarding better home care begin with this thought-provoking question to them: “Do you brush your teeth immediately after eating pretzels or potato chips?”
Both are retentive foods, which are foods that stick to your teeth. They are slow dissolving carbohydrates, and the amylase in saliva begins breaking down the starches in these snacks into sugar in their mouth! I love watching the antennas go up behind their ears because I know that I’ve suddenly captured their attention. Most of them had no idea that this was happening when they ate retentive foods for a snack.
Speech
Speaking dries the mouth. Since salivation is under nervous control, the parasympathetic impulses in nerve fibers of the facial and glossopharyngeal nerves promote the secretion of moderate amounts of saliva to keep the lips and tongue moist enough to facilitate speech. Normal speech is actually impossible without it.
Saliva’s Many Protective Functions
Dilution or elimination of sugars and other substances ‒ Saliva begins to act as a protective mechanism whenever fermentable carbohydrates, composed mainly of short-chain sugar molecules, are eaten. In its unstimulated state, saliva exists in small quantities within the mouth. As soon as any sugar is ingested, the concentration of this sugar/saliva solution rises very quickly, triggering the secretory response of the salivary glands. This creates an increase in salivary flow, causing you to swallow some of this concentrated solution of sugar.
This cycle continues as the remaining amount of sugar, perhaps along with another ingestion of more sugar, causes the sugar-saturated saliva to become concentrated again, triggering more short-term stimulation, followed by swallowing again. It is clear to see how this swallowing sequence helps to eliminate some of the newly ingested sugars along with some of the microorganisms themselves that are present in the mouth.1 Remember that sugar is a food source for the microorganisms, and once metabolized, they create acids capable of demineralizing tooth enamel and dentin.
Antimicrobial activity ‒ Saliva contains many antibacterial, antifungal, antiviral, and wound healing components. It is rich in lysozyme, which causes an enzymatic breakdown of bacterial cell walls. Some of the best antimicrobial peptides in saliva are the defensins, cathelicidins, and histatins.
Histatin-5 is a strong antifungal, primarily against the fungal pathogen Candida albicans. It helps in limiting C. albicans attachment to the oral epithelia. The oral cavity often undergoes injuries that result in wounds or ulcerations, such as biting the tongue and cheeks, trauma from dental procedures, or accidental injuries.
The wound healing capacity of the oral cavity is mainly attributed to the wound healing properties of saliva, specifically to the naturally occurring growth factors that play the most significant role in the wound healing process. One of the main growth factors found in saliva with such wound healing properties is a protein known as epidermal growth factor (EGF).11
Buffer activity (pH control) ‒ Salivary buffering (acid neutralization), elimination, and flow rate work together to regulate intraoral pH changes. The body’s salivary buffer system consists of three individual buffers (not unlike the buffer capabilities of blood): the carbonic acid/bicarbonate buffer, the phosphate buffer, and the buffering of plasma proteins. While the third buffer is the most plentiful, the carbonic acid/bicarbonate buffer system is usually considered the most important since it is coupled to the respiratory system.
The pH system ‒ We first need to review some basic concepts of the pH system to help us better understand the neutralization of mouth acids, in particular, the carbonic acid/bicarbonate buffer system. pH is a quantitative measure of the acidity or alkalinity of aqueous – or water solutions. Each unit change in pH (e.g., from a pH of 5 to a pH of 4) involves a huge, tenfold increase in acidity concentration of the hydrogen ion (H+). Further lowering the pH to 3 means a 10 times 10, or a hundredfold increase in acidity concentration from a pH of 5.
The pH scale came from the Danish chemist Søren Peder Lauritz Sørensen at the Carlsberg Laboratory in 1909. “The “H” in pH stands for hydrogen, but there is some controversy as to what “p” represents.” The Carlberg Foundation itself has said that pH means the power of hydrogen.12
The more acidic the aqueous solution, the lower the pH, and the less acidic (or more alkaline) the aqueous solution is, the higher the pH. At a pH of zero, the maximum amount of hydrogen ions is released into solution. For a pH of 14 (total alkalinity), no hydrogen ions are released into solution. The pH scale ranges from 0 to 14, which covers almost all possible situations. See Figure 4 for a pictorial description of the acidic/alkaline changes as you go through the entire range from 0 to 14.13
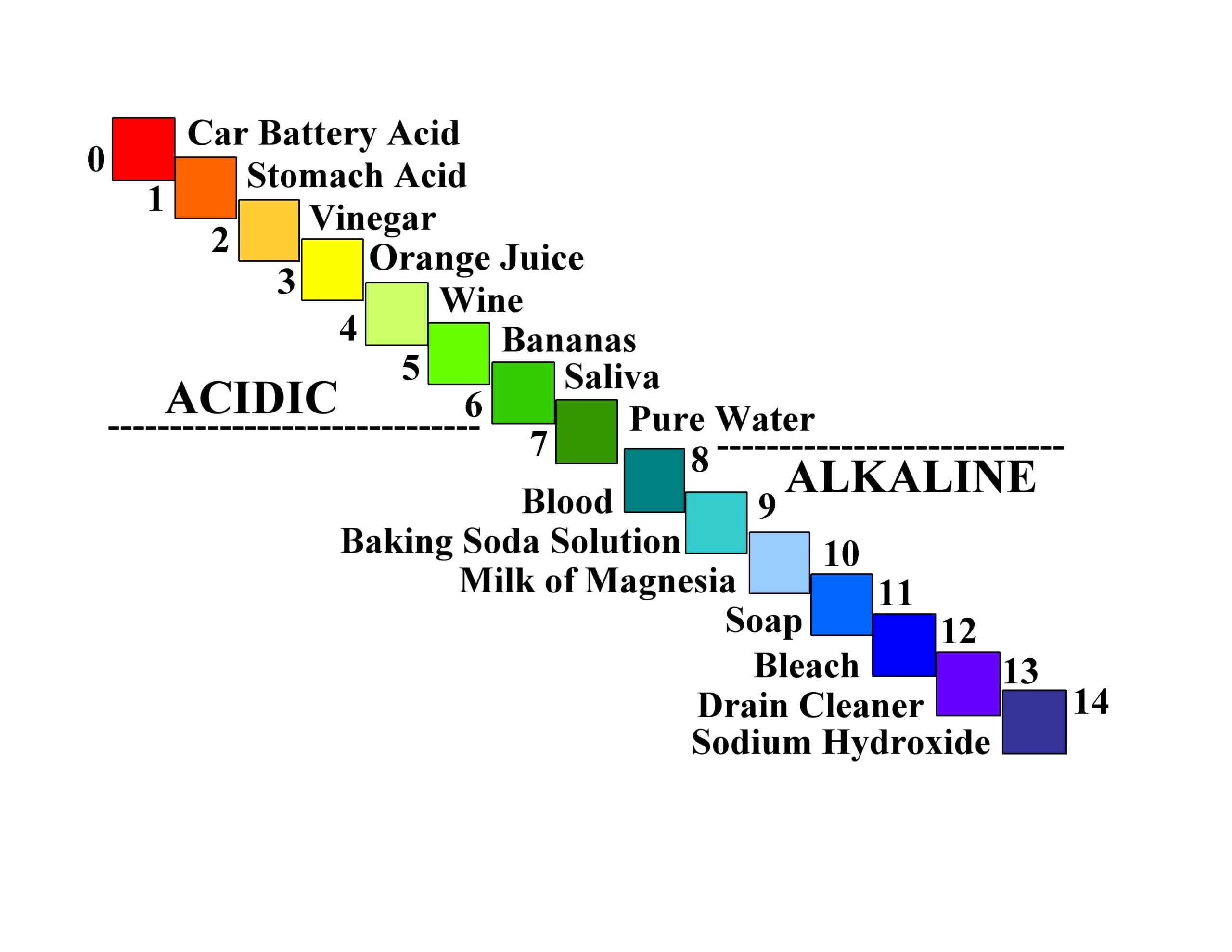
The carbonic acid/bicarbonate buffer system14 ‒ The basic chemical equation for this buffer system is:
H+ + HCO3– ⇄ H2CO3 ⇄ CO2 + H2O
(hydrogen ion + bicarbonate ion ⇄ carbonic acid ⇄ carbon dioxide + water)
Verbally, this equation tells us that the hydrogen ion (H+) can chemically combine with the bicarbonate ion, which is available in saliva. This forms a weak acid called carbonic acid (H2CO3), which can break down further into carbon dioxide and water.
When you ingest a fermentable carbohydrate, digestion begins in the mouth, causing this carbohydrate to be converted to sugar. The cariogenic bacteria living in the biofilm on a tooth metabolize the sugar and give off a relatively strong acid as a byproduct. This means that the hydrogen ion of the strong acid (H+) goes into solution in your saliva, ready to attack your tooth enamel, but the bicarbonate ions present in your saliva will readily accept this hydrogen ion from the strong acid, making carbonic acid, a weak acid. Strong acids will readily give up their hydrogen ions, and they seek to react with a negatively charged ion, such as the alkaline (negative) bicarbonate ion in your saliva.
This means that the salivary and biofilm pH values move toward a neutral pH, meaning less acidic, therefore less able to cause tooth decay. The strong acid has been buffered (neutralized), and the saliva is at its normal pH of 6.3. In addition, this weak carbonic acid formed from the reaction breaks down into water and carbon dioxide. The lungs eliminate weak acids as vapors and gases; in this case, as breath moisture and carbon dioxide.
Note, however, that damage can still occur if the acid attacks are too frequent and severe, as with many in-between snacks without you quickly clearing the debris from your mouth. Saliva would not have enough buffering capacity to neutralize this amount of acid fully.
Dental caries and the remineralization/demineralization process ‒ Dental caries is a multifactorial disease caused by the interaction between ingested fermentable carbohydrates and any biofilm on a person’s teeth. It is the cumulative result of ongoing cycles of demineralization and remineralization at the interface between the biofilm and the tooth surface. Demineralization occurs when the pH drops below the critical pH, causing dissolution of the tooth mineral hydroxyapatite. The critical pH for enamel is about 5.5, depending on the concentrations of calcium and phosphate in the biofilm.15 Because it is softer, the cementum has a critical pH ranging from 6.0 to 6.7.16 The higher critical pH means that it decays easier than enamel. Another way to explain it is: Weaker acids with a higher pH than the critical pH for enamel but less than the critical pH of cementum are not able to attack enamel, but they will attack cementum.
Many people with exposed cementum experience issues with “root caries” because of this. Normally, saliva functions as a “bathing” solution around our teeth, being strongly saturated with calcium and phosphorus. These mineral ions protect the enamel from weakening due to the acidic attacks that cause demineralization. They are ready to rebuild enamel in this constant demineralization/remineralization process.
Hydroxyapatite (HA) is chemically expressed as Ca10(PO4)6(OH)2, the chief mineral ingredient in teeth. Tooth enamel is made of over 90% HA, dentin contains between 70% to 80% HA, and cementum contains 45% to 50% HA. When in contact with saliva, the following chemical reaction occurs:
Ca10(PO4)6(OH)2 ⇄ 10Ca2+ + 6PO43− + 2OH−
Remineralization (Precipitation) ⇄ Demineralization (Dissolution)
Promoting tooth remineralization requires: (1) increasing the calcium (Ca) and phosphate (P) ion concentrations; and (2) raising the local pH where remineralization is needed.17
In demineralization, hydroxyapatite crystals are dissolved (termed dissolution) from the subsurface of the tooth (see Figure 5 – red arrow, meaning demineralization). Remineralization is the natural repair process for non-cavitated lesions. It relies on calcium and phosphate ions found in saliva to rebuild a new surface on the existing crystal remnants in the subsurface of the tooth.18 Called precipitation, it is the process of precipitating or recreating the mineral from its component ions that exist in the salivary solution.
Enamel remineralization in the presence of fluoride in biofilm ‒ Adding fluoride to a patient’s home care enhances remineralization significantly. Here’s why. When fluoride is present in saliva, fluorapatite forms during the remineralization process (see Figure 5 – green arrow, meaning remineralization) rather than hydroxyapatite.16
The formation takes place according to the following equation:
Ca10(PO4)6(OH)2 + 2F−= Ca10(PO4)6(F)2 + 2OH−
calcium hydroxyapatite (HA) + fluoride ions = calcium fluorapatite (FA) + hydroxyl ions.
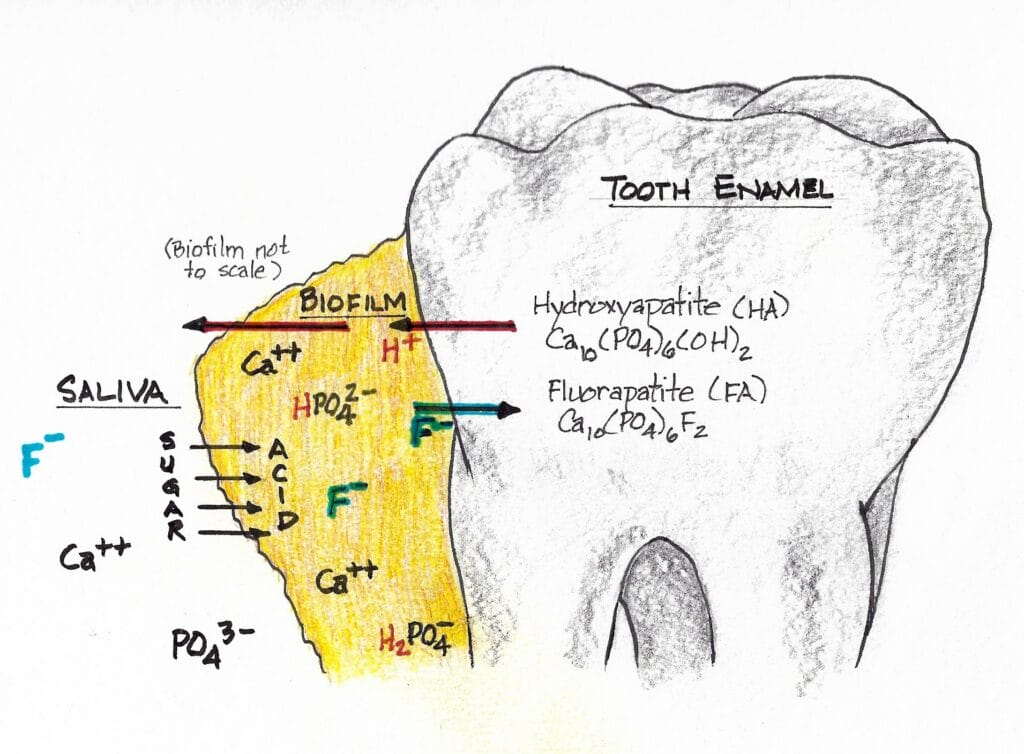
As we know, sugars are converted to acids in the biofilm. When the pH decreases below 5.5, undersaturation of hydroxyapatite (HA) is reached because we’ve gone below the critical pH of HA (5.5) in the biofilm fluid. Mineral dissolution begins.
Researchers observe, “However, if the pH is higher than 4.5 (the critical pH of FA) and fluoride is present, the biofilm fluid is supersaturated with respect to fluorapatite (FA), and there is reprecipitation of minerals in the enamel. As a consequence, the net demineralization is reduced.”19
Calcium fluorapatite is more resistant to an acid caries attack than calcium hydroxyapatite. The critical pH of hydroxyapatite is around 5.5, while that of fluorapatite is around 4.5. This is why topical fluoride treatments help patients. This varies with individual patients and is also a function of age. Below critical pH, demineralization occurs, while above critical pH, remineralization occurs.15
Think about the many foods and drinks that could make you susceptible to tooth decay if you have any cariogenic biofilm and do not use fluoride protection for your teeth! Just a few examples of common foods and drinks that have a pH between 4.5 and 5.5 (in no particular order) are black coffee, wine, beer, maple syrup, tomatoes, bananas, and pumpkins.20 This brief list may be enough of an incentive for some to begin regularly using a fluoride rinse or gel.
In Closing
We’ve studied the indispensable properties of saliva, from it being crucial in the digestive process to it helping us to speak comfortably, as well as to its many protective functions. It plays a critical role in the dilution of sugars in the mouth that promote dental caries. It contains many antibacterial, antifungal, antiviral, and wound healing components. It plays an essential role in neutralizing acids that can cause dental caries and tooth loss. We’ve also reviewed how the use of fluoride in patient home care can work together with saliva to help minimize the progression of dental caries. Labeling saliva as a miraculous fluid is a well-earned title.
Before you leave, check out the Today’s RDH self-study CE courses. All courses are peer-reviewed and non-sponsored to focus solely on high-quality education. Click here now.
Listen to the Today’s RDH Dental Hygiene Podcast Below:
References
- Puy, C.L. The Role of Saliva in Maintaining Oral Health and as Aid to Diagnosis. Med Oral Patol Oral Cir Buccal. 2006; 11(5): E449-55. https://pubmed.ncbi.nlm.nih.gov/16878065/
- Dodds, M., Roland, S., Edgar, M., et al. Saliva, A Review of its Role in Maintaining Oral Health and Preventing Dental Disease. BDJ Teams. 2015; 2: 15123. https://www.nature.com/articles/bdjteam2015123
- The Major Salivary Glands, Three Pairs in Total. (n.d.). Center for Advanced Parotid and Facial Nerve Surgery. https://www.parotidsurgerymd.com/education/articles/parotid-salivary-gland-info/
- Wakefield, R.J., & D’Agostino, M.A. (2010). Salivary Glands. In S. Jousse-Joulin, Essential Applications of Musculoskeletal Ultrasound in Rheumatology (pp. 199-206). W.B. Saunders.
- Cox, J.D., & Ang, K.K. (2010). The Salivary Glands. In A.S. Garden, Radiation Oncology: Rationale, Technique, Results (9th ed., pp. 169-182). Mosby. https://www.sciencedirect.com/science/article/abs/pii/B9780323049719000081
- Kessler, A.T., Bhatt, A.A. Review of the Major and Minor Salivary Glands, Part 1: Anatomy, Infectious and Inflammatory Processes. Journal of Clinical Imaging Science. 2018; 8: 47. https://www.ncbi.nlm.nih.gov/pmc/articles/PMC6251248/
- Salivary Glands Anatomy. (n.d.). Memorial Sloan Kettering Cancer Center. https://www.mskcc.org/cancer-care/types/salivary-gland/salivary-glands-anatomy
- Hatfield, S. (2020, October 26). Tubarial Salivary Glands: Scientists Discover New Salivary Glands in Human Throat. Today’s RDH. www.todaysrdh.com/tubarial-salivary-glands-scientists-discover-new-salivary-glands-in-human-throat/
- Valstar, M.H., de Bakker, B.S., Steenbakkers, R.J.H.M., et.al. The Tubarial Salivary Glands: A Potential New Organ at Risk for Radiotherapy. Radiology and Oncology. 2021; 154: 292-298. https://www.sciencedirect.com/science/article/pii/S0167814020308094
- Iogulescu, G. Saliva Between Normal and Pathological, Important Factors in Determining Systemic and Oral Health. Journal of Medicine and Life. 2009; 2(3): 303-307. https://www.ncbi.nlm.nih.gov/pmc/articles/PMC5052503/
- Vila, T., Rizk, A.M., Sultan, A.S. The Power of Saliva: Antimicrobial and Beyond. PLOS Pathogens. 2019; 15(11): e1008058. https://www.ncbi.nlm.nih.gov/pmc/articles/PMC6855406/
- Bradley, D. (2018, February 21). When It Comes To Caustic Wit and an Acid Tongue; Mind your Ps and Qs. Materials Today. https://www.materialstoday.com/materials-chemistry/comment/caustic-wit-acid-tongues-mind-your-ps-and-qs/
- Reddy, A., Norris, D.F., Momeni, S., et al. The pH of Beverages Available to the American Consumer. J. Am. Dent. Assoc. 2016; 147(4): 225-263. https://www.ncbi.nlm.nih.gov/pmc/articles/PMC4808596/
- Stefan, V. (2015, July 18). What Is the Carbonic Acid-bicarbonate Buffer System? Socratic Q&A – Chemistry. https://socratic.org/questions/what-is-the-carbonic-acid-bicarbonate-buffer-system-1
- Goldstep, F. (2012, December 1). Dental Remineralization: Simplified. OralHealth. https://www.oralhealthgroup.com/features/dental-remineralization-simplified/
- Wilkins, E.M. (2017). Clinical Practice of the Dental Hygienist (12th ed.). Jones & Bartlett Learning.
- Liang, K., Wang, S., Tao, S., et al. Dental Remineralization Via Poly (Amido Amine) and Restorative Materials Containing Calcium Phosphate Nano Particles. International Journal of Oral Science. 2019; 11(15). https://www.nature.com/articles/s41368-019-0048-z
- Neel, E.A.A., Ajabo, A., Strange, A., et al. Demineralization – Remineralization Dynamics in Teeth and Bone. Int J Nanomedicine. 2016; 11: 4773-4763. https://www.ncbi.nlm.nih.gov/pmc/articles/PMC5034904/
- Cury, J.A., Tenuta, L.M.A. Enamel Remineralization: Controlling the Caries Disease or Treating Early Carious Lesions? Brazil Oral Res. 2009; 23(Spec lss 1): 23-30. https://www.scielo.br/j/bor/a/X3cB43Z7gfrwnmHnthX5gdp/?lang=en&format=pdf
- pH Value of Common Foods and Ingredients. (n.d.). Clemson University. https://www.clemson.edu/extension/food/food2market/documents/ph_of_common_foods.pdf